How to Design a Gene Therapy to Target Viruses
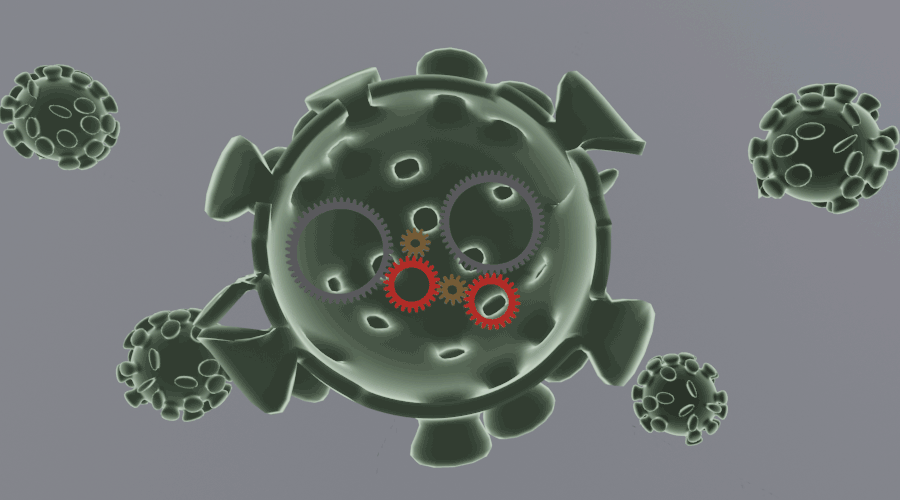
This is a lesson you won’t learn in school. We’re at an exciting stage in biotechnology today, on the cusp of revolutionizing how we treat some of humankind’s most deadly diseases. Read on to learn how new gene therapy techniques are ushering a new age of antiviral medicine.
How a virus works
Viruses are odd. They have a distinct and sophisticated genetic identity, but no way to affect the world around them unless they can get into a host and hijack its cellular machinery, turning it into a viral factory. These curious members of the web of life are assembled like mechanical wristwatches inside the cell: each viral gene is a blueprint to create a viral protein, and those proteins are like the gears and plates that make the watch tick. All of the pieces need complementary shapes to mesh together and function harmoniously with the other viral proteins, and interact with the host’s machinery. Although viruses only carry a handful of essential genes, they are able to mesh with the cell and direct it to perform intricate tasks such as de-regulating the cell, assembling viruses efficiently, and avoiding the immune system. With so many things that need to go right, interrupting any of these steps can bring the infectious cycle to a halt.
Traditional pharmaceuticals typically inhibit virus replication with a small molecule; like dropping a little grain of sand into the gears of the wristwatch. Unfortunately, viruses mutate so quickly that generation over generation, they can morph and develop resistance to these small molecules. Given how fast they replicate, resistance can be developed very quickly, so for viruses like HIV, multiple antivirals are often combined – bringing replication to a standstill so the viruses cannot prototype and innovate. Viruses like HIV, that hide in the nucleus tend to be the viruses that keep people infected for life, like members of the herpesvirus family, meaning that traditional medicines need to be taken for life too. Surely there’s a better way than a pill a day.
Gene therapies target the virus at the genetic level
Against a replicating, mutating foe that needs constant attention, traditional medicines can only pause the problem. But a new class of medicine may be able to solve the problem.
Gene therapies are able to address the virus right at its very identity on the genetic level. CRISPR, RNA interference, viral vectors and other tools can be pieced together to edit, inhibit, or deliver genes, respectively. These tools open the possibility for entirely new approaches to counter viruses. Instead of jamming the “gears” of a virus with a small molecule, gene therapy opens the possibility to remove a gear altogether.
So, now that we have these powerful tools, how can we apply them to cure a viral infection?
Block the most critical genes of the virus
The first step to curing a virus is to learn everything you can about the species you’re targeting. Once you understand how the virus copies itself, how it gets into cells, and any other features that make it difficult for the body to handle, you can start isolating the most important viral genes involved in its lifecycle.
Finding genes which don’t change much from virus to virus is a good indication that they’re important and that they can’t mutate much before they lose their function. The best genes to target for removal/inhibition with gene therapy are the ones which are critical to viral replication and can’t afford to change too much.
Once you find 1-3 genes to target, the next step is to choose the best gene therapy tool to suppress your targets.
The biotechnology toolset: CRISPR and RNA interference
These days, most people have heard about CRISPR-Cas9, the molecular scissors which can be guided to a specific sequence of DNA. The discovery of the CRISPR system rocketed gene editing to the public consciousness, and researchers have been able to generate massive amounts of data by easily swapping out the RNA that guides these scissors, changing which genes are targeted.
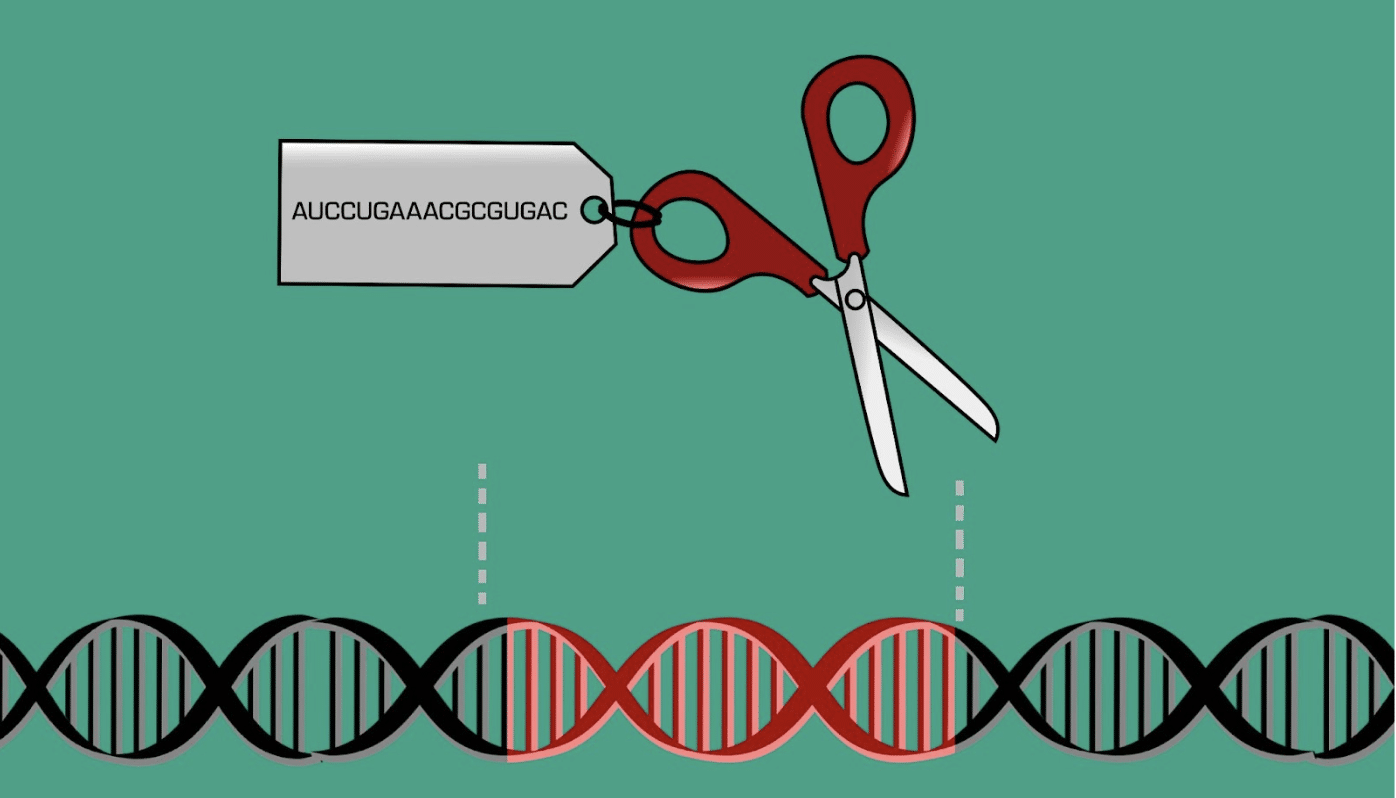
By lining up a sequence of approximately twenty complementary nucleotides so that the AUGCs in the guide RNA match up to the ATGCs in the genome, the scissors can precisely target unique sequences of DNA. However, cutting DNA can harm the cell in some cases, particularly if there was an unidentified role associated with the stretch of DNA being edited. If the DNA is repaired perfectly, it may be recognized by the scissors again,and repeated cuts will eventually result in a mutation through imperfect DNA repair. This is why many gene therapies being developed for human use rely on RNA interference instead of CRISPR/Cas9 systems.
Unlike CRISPR/Cas9 systems which are adapted from prokaryotes (mostly bacteria), RNA interference is a mechanism native to humans that intercepts specific mRNAs, and blocks them from being turned into proteins. The master copy of the gene is written in DNA, but in order for that gene to affect the cell, an short-lived RNA copy is produced and then consumed to affect the cell's behavior. Human cells use RNA interference to intercept and destroy these temporary RNAs before they can affect the cell’s behavior, decreasing or completely eliminating the effect of specific genes. By understanding how human cells use RNA interference and adding to their capabilities, scientists can block specific genes (like viral genes) from being expressed without risking off-target cuts or DNA mutations characteristic of CRISPR/Cas9 systems.
There are multiple mechanisms of RNA interference which can selectively target viral mRNA, but no matter which mechanism a researcher chooses to use, it’s best to give the cell a master copy of the instructions so that it can always be on the lookout for viral messages. Delivering this “update” to the pre-existing RNA interference machinery can be done by introducing a new gene to the cell. To do this, we can package the “update” into an empty virus shell, formally known as a viral vector. The shell is like a virus’ targeting system and it can dissolve after it delivers its cargo, making it a one-way delivery system. Once the cell has its new instructions, you can test how well the antiviral RNA interference gene works by placing some cells in a petri dish and introducing a real, infectious virus. If the antiviral gene works, the introduced virus will not be able to replicate due to the new obstacles in its way, and it’s time to move on to advanced stages of testing.
Gene therapies can usher in a new age of antiviral treatments
There are viral infections that can remain with a person for their entire life, and traditional medicine could only stop them from replicating if the patient was constantly taking medication. With gene therapy, it is possible to stop viruses in new ways, giving the cell the tools it needs to recognize and block viral messages. Using today’s methods, it’s possible to educate cells on how to impede viruses instead of relying on constant doses of external medication. Today’s biological tools allow us to target viruses which were previously thought of as incurable. In fact, Montgomery County Maryland’s American Gene Technologies® is using methods like the ones described here to pursue cures for infectious and rare diseases.
The computer revolution provided the power necessary to crunch through mountains of raw genomic data and understand the function of genes. Now, modern biological techniques allow us to design and deliver updates to cells, written in DNA, that address viruses right at the source. This is how the age of information seamlessly flows into the age of biology, and this is how modern medicine may finally cure the incurable. If you can dream it, you can do it – and the next generation of biological researchers will undoubtedly use these tools to imagine cures for myriad conditions.
Find out more about what American Gene Technologies is doing to revolutionize gene therapy.